Evaluation of various constellations and detail studies on specific subjects
In order to study the impact of different values for the period of a repeat orbit or (sub)cycle and the impact of a change of height, several scenarios were defined for Bender-type constellations consisting of one pair flying in a (near-)polar orbit and one pair in an inclined orbit (see Table 1). In addition, a few sun-synchronous orbital (SSO) and pendulum missions were defined. The nominal baseline length is equal to 220 km for all satellite pairs and scenarios. For the scenarios 3d_H and 5d_LL also pendulum pairs with angles of 150, 300, and 450 were defined.
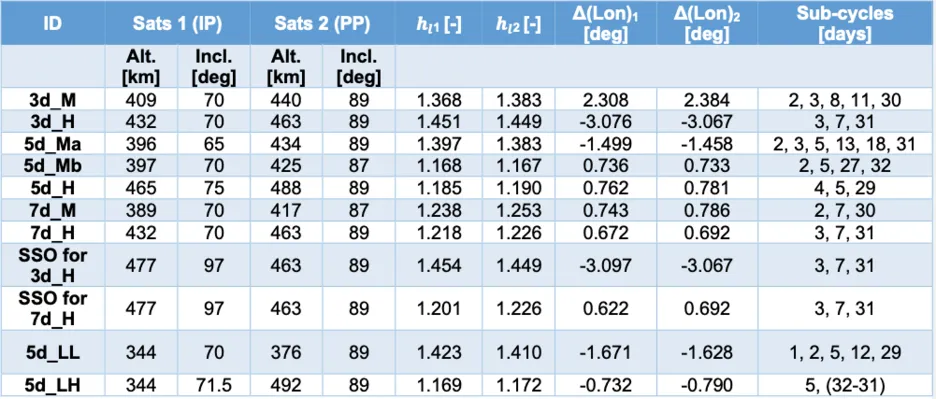
These results, which are mainly based on the 3d_H scenario including realistic error models for the key instruments and tidal and non-tidal background errors, clearly demonstrate the superior performance of Bender double-pair mission concepts over all other potential constellations, such as single-pair inline or pendulum missions. There is no added value by flying a pendulum pair as part of a Bender-type constellation. Also, the concept of a chronometric 3-satellite pendulum mission was ruled out by these results. In general, temporal aliasing errors are the dominant error source. However, an improved accelerometer (~10-11 m/s2sqrt(Hz)) has relatively higher impact in double pair constellations (due to improved de-aliasing capabilities).
Among others, detailed numerical studies were also performed regarding the following aspects:
a) Dependence of performance on the altitude of the satellites/satellite pairs
It could be confirmed that the altitude is the main performance driver. Since in a Bender double-pair scenario the relative contribution of the inclined pair to the total performance is more than 90% in the areas covered with measurements, a low altitude together with a high-performance instrumentation of the inclined pair is essential.
b) Optimum inter-satellite distance
The choice of the inter-satellite distance is a compromise between sensitivity (which improves with distance) and spatial resolution (which degrades with distance). As optimum, an inter-satellite distance of 200-250 km is recommended.
c) Double-pair: sensitivity w.r.t. inclination of inclined pair
A rather low inclination of second pair (≤70°) is paramount for the de-aliasing capability of the constellation. Raw (unfiltered) 70° solutions are more stripy (about 50%) than 65° solutions in the covered areas, due to worse estimates of (near-)sectorials, but are better (by about 50%) in the polar areas, due to smaller polar gaps and better estimates of the polar wedge. Post-processed (filtered) 65° solutions are on global average up to 10% better than 70° solutions. This holds for both covered and polar regions. However, this percentage numbers vary with the choice of the filter. An obvious severe disadvantage of 65° inclined orbit is that it results into a larger unobserved region near the poles as stand-alone mission. Therefore, a more conservative 70° inclination of the inclined pair is recommended.
d) Impact of ground track sampling on gravity performance and heterogeneity
Figure 2 shows the simulation set-up for a 3-day solution using polar and inclined orbits with 3-day and/or 5-day sub-cycles polar and inclined orbit (mixture of orbits of the scenarios given in Table 1.), leading to homogeneous coverage (3d_H), spatial gaps of the polar tracks (U3d5d_H) or gaps in both pairs (U5d_H). Evidently, a non-homogeneous ground track pattern of the polar pair (blue curve in Figure 3) is acceptable, but leads to slightly larger errors in higher spherical harmonic (SH) degrees compared to the homogeneous ground track sampling of both pairs (red curve). However, combining a polar pair having a non-homogeneous ground track pattern with an inclined pair also having a non-homogeneous ground track pattern with coinciding gaps in the 3-day ground tracks of both pairs results in a severe degradation over the whole spectral range, and thus hampers significantly the homogenous quality especially of short-term (NRT) solutions required for operational service applications.
e) Impact on drag-free conditions and accelerometer performance
Various scenarios representing a combination of the MicroSTAR instrument performance combined with different levels of imperfect drag compensation for the inclined satellite pair in regard to different atmospheric conditions, ranging from a “best-case” full 3D (blue curve in Figure 4) to a “worst-case” 1D drag compensation in along-track direction and with maximum atmospheric conditions (red curve), have been analyzed. In all cases, the same assumption on the polar pair of a SuperSTAR ACC is applied. Figure 5 a shows, that in the product-noise only case, a degradation of varying extent of all scenarios compared to the reference scenario (black curve) is visible, with the maximum impact up to degree/order 30. It should be emphasized, that a stochastic modelling of the tone error peaks at multiples of the orbital frequency (cf. Figure 4) as part of the gravity adjustment process is indispensable to avoid significant degradation of the retrieval performance. In case of the full-noise simulation scenario (Figure 5 b) where de-aliasing is applied for the AO and OT components with the HIS component as target signal, the differences between the investigated scenarios are much smaller, because most of the impact of the drag compensation is covered by the temporal aliasing.

f) Scaling of performance with retrieval period
As to be expected, in the product-noise only case the performance can be scaled linearly with the number of observations (= retrieval period). We found out that this linear scaling can also be roughly applied to the full-noise solutions (including background model errors) down to about 3 days. In contrast, co-estimated as well as stand-alone daily solutions perform even better than predicted by linear scaling.
g) Capabilities of short-term (near-real time) retrieval
Short-term daily co-estimates (proposed by Wiese et al. 2011) are possible up to SH degree 15 to 20 for double-pair scenarios. In specific cases this parameterization causes “bumps” in the degree variance plots at higher degrees. This happens whenever the inclined pair has a very high weighting compared to the polar pair, for example if it has a significantly lower altitude or a much better instrument (accelerometer) performance. In this case, alternatives to the classical “Wiese parameterization” will have to be applied, such as the DMD method (cf. section 4 c).
h) Raw vs. post-processed (filtered) solutions, effect of filtering
Depending on the post-processing strategy, a wide range of results can be achieved in comparison to the MRD/IUGG requirements. It is recommended to use the raw (unfiltered) solution as the baseline strategy for the evaluation of mission performances, keeping in mind that this composes the “worst case” compared to the IUGG requirements, which assumed a certain degree of post-processing.
i) Effect of tone errors
The effect of tone errors is clearly visible in product-only cases, where mainly the low-degree zonal SH coefficients are affected. An adequate stochastic modelling of tone errors is paramount to avoid significant degradation of higher-degree coefficients. Increasing the amplitude of tone errors by a factor of 10 w.r.t. the original SRD specifications, their impact reaches the error level of the full-noise solution, where the effect of tone errors is partly mixing with other error sources. Based on these results, it was recommended to relax the SRD requirements regarding tone error amplitudes by a factor of 10.